Introduction
While Hg can be determined by Inductively Coupled Plasma Optical Emission Spectrometry, (ICP-OES) with conventional pneumatic nebulization, the low μg/L detection limits typically achieved are not adequate for most environmental applications. The Cold Vapor technique, using an acidified stannous chloride reductant (SnCl2) solution, is a widely-used technique to improve the sensitivity of Hg in atomic spectroscopy. The Cold Vapor process traditionally uses a dedicated instrument, or a Cold Vapor/Hydride Generation accessory connected to the ICP. However, for laboratories that routinely determine Hg and other elements in the same sample, switching between a cold vapor and conventional nebulization modes adds complexity, increases argon (Ar) consumption and reduces laboratory productivity.
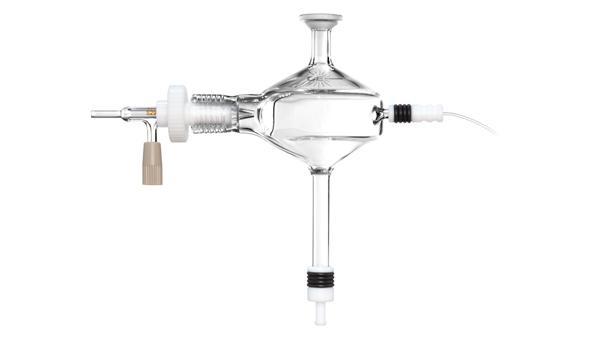
Glass Expansion, world leaders in sample introduction systems for ICP-OES and ICP-MS have made a single-pass cyclonic spray chamber with conventional pneumatic nebulization capabilities and a secondary inlet port to inject a stream of SnCl2 into the nebulizer aerosol plume. The aerosol/liquid interaction ensures a rapid mixing of the aerosolized Hg in the nebulizer plume with the acidified SnCl2, efficiently converting Hg into a volatile form to improve Hg sensitivity. In this simple arrangement, the HydraMist gave a 20-fold improvement in Hg detection limits compared to conventional pneumatic nebulization setup, without degrading the precision or sensitivity of the other elements being conventionally nebulized through the SeaSpray nebulizer.
Instrument
The ICP-OES used in this work was an Agilent Technologies® 5100 SVDV ICP-OES, with a HydraMist spray chamber and Glass Expansion SeaSpray nebulizer with operating conditions indicated in Table 1 below. To compare the performance of the system without the Cold Vapor process the analysis was repeated using a standard Tracey single-pass spray chamber from Glass Expansion instead of the HydraMist spray chamber.
Experimental Parameter | Setting |
RF power | 1.4kW |
Nebulizer gas flow rate | 0.60 L/min |
Plasma gas flow rate | 12 L/min |
Auxillary gas flow rate | 1.0 L/min |
Real-time | 30 sec |
Number of replicates | 3 |
Peristaltic pump speed | 20 rpm |
Stabilization time | 30 sec |
Sample line pump tubing | White/white |
SnCl2 line pump tubing | Black/black |
Drain tubing | Black/white |
Nebulizer | 2 mL/min Direct Connection SeaSpray |
The instrument was operated at a constant sample uptake with the option of the fast rinse between samples disabled.
As SnCl2 can form an insoluble salt when dissolved in water, it is usually dissolved in concentrated HCl acid first and then diluted with H20 to form a 5% SnCl2 solution in 5% HCl.
The sample was Standard Reference Material 1643f Trace Elements in Water from the National Institute of Standard Technology (NIST) Gaithersburg, MD. SRM 1643f does not naturally have detectable Hg so it was spiked with 5ppb for this work.
Results & Discussion
As can be seen in Figure 2, the optimum concentration of SnCl2 reaches a maximum of about 5% and plateaus after that.

In Figure 3, the intensity of Hg at 194nm is measured as a factor of sample uptake rate. The sample uptake rate is adjusted by varying the instrument peristaltic pump speed. The intensity of Hg increases as the volume of the sample aspirated is increased, indicating the Cold Vapor process is sample-limited, not reductant limited.
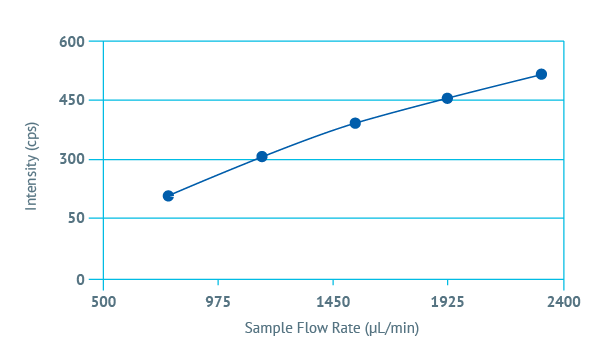
In Figure 4, the Signal to Root Background Ratio (SRBR) and Net Intensity of Hg at 194nm was evaluated as a function of nebulizer flow. The nebulizer gas flow not only aspirates the analyte solution but it is also used to transport the sample aerosol and Hg Cold Vapor into the plasma. SRBR is used to optimize nebulizer flow, as it is a good proxy for detection limit performance in a solid-state detector-based ICP-OES. As can be seen in Figure 4, the optimum nebulizer flow rate is around 0.6 L/min Ar.
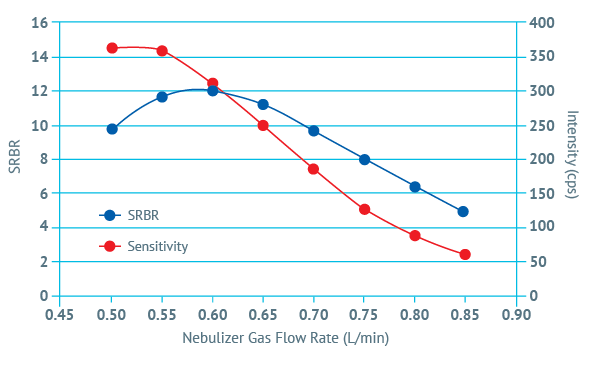
Figure 5 shows a comparison of blank intensity, and 5ppb and 10ppb Hg intensities with and without SnCl2 showing Hg sensitivity enhancements due to cold vapor generation using this SnCl2 - HydraMist method.
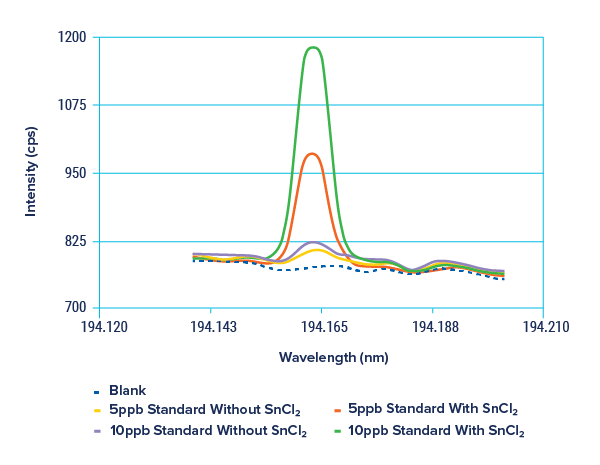
Table 2 presents 3σ Detection Limits (ppb) comparison between HydraMist and Tracey spray chambers.
Analyte λ (nm) |
HydraMist 3σ Detection Limits (ppb) |
Tracey 3σ Detection Limits(ppb) |
As 188
|
2.3 | 2.5 |
Be 313
|
0.001 | 0.01 |
Cd 214
|
0.1 | 0.1 |
Co 233 | 0.6 | 0.6 |
Cr 268 | 0.3 | 0.3 |
Cu 327 | 0.7 | 0.5 |
Hg 194 | 0.2 | 4.2 |
Mn 257 | 0.03 | 0.03 |
Mo 202 | 0.5 | 0.6 |
Ni 232 | 0.6 | 0.8 |
Pb 220 | 2.1 | 2.4 |
Sb 217 | 2.8 | 2.6 |
Se 196 | 3.6 | 3.5 |
Tl 191 | 2.4 | 2.4 |
V 292 | 0.4 | 0.4 |
Zn 231 | 0.2 | 0.2 |
The measured concentration of 16 elements in SRM 1643f “Trace Elements in Water” using the HydraMist spray chamber and SeaSpray nebulizer under the conditions listed in Table 1 are shown in Table 3 below. As the SRM does not contain measurable concentrations of Hg, a 50 mL aliquot of the SRM was spiked with 25 μL of 10 ppm Hg, for a 5 ppb spike concentration.
Analyte λ (nm) |
SRM 1643f Found (ppb) |
SRM 1643f Certified (ppb) |
Recovery % |
As 188 | 60.5 | 57.4 | 105 |
Be 313 | 13.6 | 13.7 | 100 |
Cd 214 | 5.8 | 5.9 | 99 |
Co 233 | 25.5 | 25.3 | 101 |
Cr 268 | 18.5 | 18.5 | 100 |
Cu 327 | 22.9 | 21.7 | 106 |
Hg 194 | 5.3* | 5.0 | 106 |
Mn 257 | 37.1 | 37.1 | 100 |
Mo 202 | 121.9 | 115.3 | 106 |
Ni 232 | 65.1 | 59.8 | 109 |
Pb 220 | 19.0 | 18.5 | 103 |
Sb 217 | 56.3 | 55.5 | 102 |
Se 196 | 11.9 | 11.7 | 103 |
TI 191 | 6.3 | 6.9 | 91 |
V 292 | 37.3 | 36.1 | 103 |
Zn 231 | 74.8 | 74.4 | 101 |
The 5.3 ppb measured value for the 5 ppb Hg spike, represents a 106% recovery using the cold vapor mode of the HydraMist spray chamber. The measured values of all 15 naturally occurring elements were within 10% of the certified values, indicating the HydraMist spray chamber is not only sensitive for Hg by cold vapor, but also suitable for measuring trace elements in waters using conventional pneumatic nebulization mode.
Conclusion
When using the Agilent Technologies® 5100 SVDV ICP-OES with HydraMist spray chamber in cold vapor mode, the 3σ detection limits of Hg were found to be 0.2 μg/L compared to the 4.2 μg/L detection limits with a conventional Tracey single-pass spray chamber. The measured results for the “naturally occurring” elements and the 5 μg/L Hg spike in SRM 1643f Trace Elements in Water were all found to be within 10% of the expected values, demonstrating the HydraMist spray chamber is a simple and sensitive sample introduction system suitable for the simultaneous detection of Hg using Cold Vapor and other trace elements by conventional pneumatic nebulization.